PROTEIN DIGESTION & AMINO ACID ABSORPTION
- Proteolytic enzymes (proteases) degrade dietary proteins into their constituent amino acids in the stomach and intestine.
- Digestive proteases are synthesized as larger, inactive forms (zymogens), which, after secretion, are cleaved to produce active proteases. The amino acids indicated after the curly brace in the diagram below are the preferred amino acids at which each of the indicated enzymes cleaves.
- In the stomach, pepsin begins the digestion of dietary proteins by hydrolyzing them to smaller polypeptides.
- Pepsinogen is secreted by chief cells of the stomach, parietal cells secrete HCl. The acid environment alters the conformation of pepsinogen so that it can cleave itself to yield pepsin.
- Pepsin acts as an endopeptidase to cleave dietary proteins with a broad spectrum of specificity, although it prefers to cleave peptide bonds in which the carboxyl group is provided by aromatic or acidic amino acids. The products are smaller peptides and some free amino acids
- In the intestine, bicarbonate neutralizes stomach acid, and the pancreas secretes several inactive proenzymes (zymogens), which, when activated, collectively digest peptides to single amino acids.
Enteropeptidase, secreted by the brush border cells of the small intestine cleaves trypsinogen to yield the active serine protease trypsin.
- Trypsin cleaves inactive chymotrypsinogen to yield active chymotrypsin, inactive proelastase, to yield active elastase, and inactive procarboxypeptidases to yield active carboxypeptidases. Thus, trypsin plays a central role because it cleaves dietary proteins and activates other proteases that cleave dietary protein.
- Each protease exhibits cleavage specificity: trypsin cleaves at the carboxy side of arg and lys; chymotrypsin cleaves at the carboxy side of phe, tyr, trp and leu; elastase cleaves at the carboxy side of ala, gly and ser. Carboxypeptidase A cleaves single amino acids from the carboxyl terminus, with a specificity for hydrophobic and branched side chain amino acids; carboxypeptidase B cleaves single amino acids from the carboxyl terminus, with a specificity for basic (arg and lys) amino acids.
- Aminopeptidases, located on the brush border, cleave one amino acid at a time from the amino end of peptides.
- Intracellular peptidases cleave small peptides absorbed by cells.
- Amino acids are absorbed by intestinal epithelial cells and released into the blood.
Click The Image
Amino Acid Transport Proteins
Amino acids are absorbed from the intestinal lumen by a group of transporters on the apical surface of enterocytes that couple their transport to the transport of Na+ ions. At least seven different carrier proteins transport different groups of amino acids across the enterocyte plasma membrane.
CO-Transport Of Sodium
The co-transport of Na+ from the outside of the apical membrane to the inside of the cell is driven by the low intracellular Na+ concentration.
The Sodium-Potassium ATPase
The low intracellular concentration of Na+ is maintained by the sodium-potassium ATPase on the basal-lateral membrane, which actively transports the Na+ out of the cell in exchange for K+. This transport maintains a Na+ gradient, which allows the cells to concentrate amino acids from the intestinal lumen.
Facilitative Amino Acid Transport
Amino acids leave the enterocyte by a facilitative transporter on the basal-lateral surface of the enterocyte for entry into the blood.
- The sodium-amino acid carrier system involves the uptake by the cell of a sodium ion and an amino acid by the same carrier protein (cotransporter) on the luminal surface of the intestine. There are at least seven different carrier proteins that transport different groups of amino acids. The sodium ion is pumped from the cell on the serosal side (across the basolateral membrane) by the Na+ - K+ ATPase in exchange for K+, providing the driving force for transport of amino acids into the intestinal epithelial cells. The amino acid travels down its concentration gradient into the portal blood, crossing the basal epithelial membrane via a facilitated transporter. Genetic defects in genes encoding the carrier proteins can result in abnormal amino acid uptake from the intestines, leading to amino acid deficiency (e.g. Hartnup disease, in which neutral amino acids are neither transported normally across the intestinal epithelium nor reabsorbed normally from the kidney glomerular filtrate, leading to hyperaminoacidurea; hypercystinurea, high urine cysteine, occurs with a frequency of approximately 1 per 7000 live births worldwide and may cause renal caliculi - kidney stones).
- Amino acids enter cells from the blood principally by Na+-dependent cotransporters and, to a lesser extent, by facilitated transporters. The Na+-dependent transport in liver, muscle, and other tissues allows these cells to concentrate amino acids from blood. These transport proteins are encoded by different genes and have different specificities than those encoded by the genes specifying the luminal membrane amino acid transporters of the intestinal epithelia. They also differ somewhat between tissues (e.g., the transport system for glutamine uptake present in liver is either not present in other tissues or is present as an isoform with different properties).
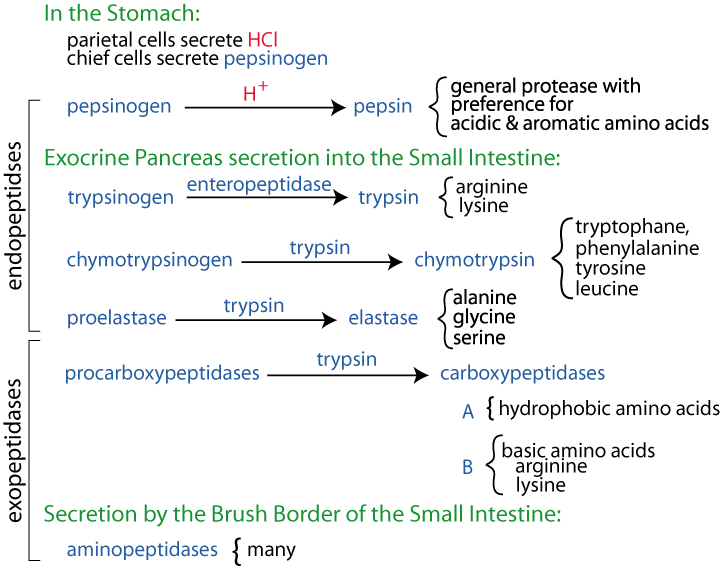
Click The Image
Protein Meal
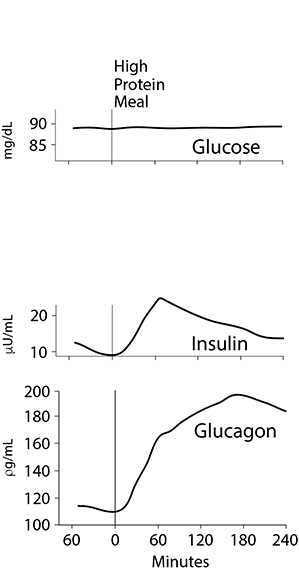
Blood levels of Glucose, Insulin, Glucagon
Carbohydrate Meal

Blood levels of Glucose, Insulin, Glucagon
A High Protein Meal
Following ingestion of a high protein meal, the gut and the liver utilize most of the absorbed amino acids. Glutamate and aspartate are utilized as fuels by the gut, and very little enters the portal vein. The gut may also use some branched chain amino acids. The liver takes up 60 - 70% of the amino acids present in the portal vein. These amino acids, for the most part, are converted to glucose.
After a pure protein meal, the increased levels of dietary amino acids reaching the pancreas stimulate the release of glucagon above fasting levels, thereby increasing amino acid uptake into the liver.
- glucagon causes increased expression of amino acid transporters on the liver cell surface
- amino acids are deaminated in the liver and carbon skeletons are used for gluconeogenesis
- urea production increases to eliminate the increased nitrogen
- arginine is a positive regulator of the first enzyme of the urea cycle, carbamoyl phosphate synthetase I by stimulating the synthesis of N-acetyl glutamate, which in turn, stimulates carbamoyl phosphate synthetase I (see Nitrogen > Urea Cycle in the menu).
Insulin release is also stimulated, but not nearly to the levels found after a high carbohydrate meal (figure at the right; click the image for a comparison with a carbohydrate meal and note the differences in the blood glucose, insulin and glucagon levels between a low or no carbohydrate protein meal and a high carbohydrate meal). In general, the insulin released after a high protein meal is sufficiently high that net protein synthesis is stimulated, but gluconeogenesis in the liver is not inhibited. The higher the carbohydrate content of the meal, the higher the insulin/glucagon ratio and the greater the shift of amino acids away from gluconeogenesis into biosynthetic pathways in the liver, such as the synthesis of plasma proteins.
Most of the amino acid nitrogen entering the peripheral circulation after a high protein meal or a mixed meal is present as the branched chain amino acids (leucine, isoleucine, valine). Because the liver has low levels of transaminases for these amino acids, it cannot oxidize them to a significant extent and they enter the systemic circulation. The branched chain amino acids are taken up slowly by skeletal muscle and other tissues. These peripheral non-hepatic tissues utilize the amino acids derived from the diet principally for net protein synthesis.