DIGESTION, ABSORPTION, AND TRANSPORT OF
THE MAJOR DIETARY CARBOHYDRATES
Carbohydrates comprise approximately 40 to 45% of the caloric intake in the average western diet. The major carbohydrates consumed are starches, sucrose, maltose and lactose. Plant starches, polysaccharides containing tens of thousands to a million glucosyl units, comprise approximately 50 to 60% of the carbohydrate calories consumed. 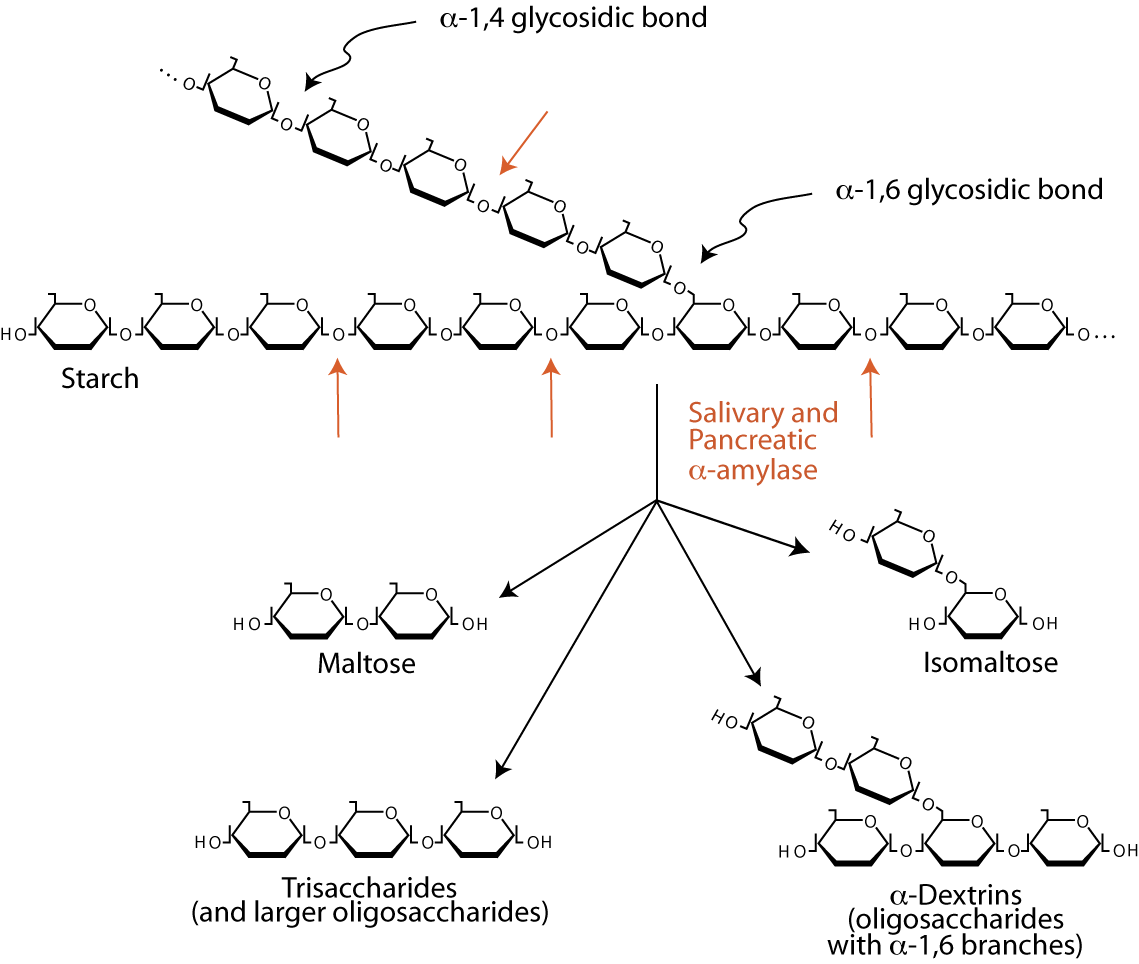
Dietary polysaccharides and disaccharides are converted in the digestive tract to monosaccharides by glycosidases and then enter the bloodstream. Undigested carbohydrates enter the colon, where they may be fermented by bacteria.
The digestion of starch beings in the mouth by the action of salivary α-amylase, an endoglucosidase that hydrolyzes internal α-1,4 bonds between glucosyl residues at random intervals to yield shortened polysaccharide chains called α-dextrins. Salivary α-amylase is largely inactivated by the acidity of the stomach. The exocrine pancreas secretes bicarbonate, which neutralizes the acid, and digestive enzymes into the duodenum, including pancreatic α-amylase, which continues to hydrolyze starches and glycogen to the disaccharide maltose, the trisaccharide maltotriose and oligosaccharides called limit dextrins of between four and nine glucosyl residues. The two glucosyl residues linked by α-1,6 bonds eventually become isomaltose. α-amylase has no activity against α-1,6 glycosidic bonds and little activity against α-1,4 bonds at the reducing end of the chain.Complex | Catalytic Sites | Principal Activities |
Glucoamylase | α-Glycosidase * | Splits α-1,4 glycosidic bonds between glycosyl units, beginning sequentially with the residue at the nonreducing end of the chain (an exoglycosidase) |
Sucrase-Isomaltase | Sucrase-Maltase | Splits sucrose, maltose (disaccharides) and maltotriose (trisaccharide) |
Isomaltase-Maltase | Splits α-1,6 bonds in a number of limit dextrins, as well as the α-1,4 bonds in maltose and maltotriose | |
β-Galactosidase | Lactase | Splits the β-1,4 bonds between glucose and galactose |
Glucosyl-Ceramidase | Splits β-glycosidic bonds between gluucose or galactose and hydrophobic residues, such as glycosylceramide and galactosylceramide (glycolipids) |
*α-Glycosidase is targeted by the drugs Acarbose, Miglitol, Vogliobose to treat type 2 diabetes by inhibiting the degradation of polysaccharides, which are not absorbed from the intestine, thereby reducing the amount of monosaccarides that enter the blood and, thus, lowering the amount of blood carbohydrates.
Several intestinal brush border glycosidases digest the products of α-amylase to monosaccharides that can be transported into the enterocytes that line the small intestine and subsequently into the blood (absorption).
The disaccharide sucrose, and small amounts of the monosaccharides glucose and fructose are the major natural sweeteners in fruit, honey and vegetables. Most foods derived from animals, such as meat or fish, contain little carbohydrate except for a small amount of glycogen. The major dietary carbohydrate of animal origin is lactose, a disaccharide of glucose and galactose, present in milk and milk products. Sucrose is hydrolyzed to its monosaccharide components, glucose and fructose, by the sucrase-maltase catalytic site of the sucrase-isomaltase complex, which also has maltase activity (the isomaltase-maltase site of the complex accounts for almost all of the intestine’s ability to hydrolyze α-1,6 glycosidic bonds, as well as maltose). This complex account for 80% of the maltase activity of the small intestine, the remainder being present in the glucoamylase complex.
Lactose is hydrolyzed to its component monosaccharides, galactose and glucose, by the lactase catalytic site of the
β-galactosidase complex (the major activity of the other catalytic site in humans is the hydrolysis of the β-bond between glucose or galactose and ceramide in glycolipids, which is hydrolyzed by the glucosyl-ceramidase). Lactose intolerance (hypolactasia) is a condition of pain, nausea, and flatulence after the ingestion of foods containing lactose, most notably dairy products, and results from insufficient lactase. Lactase activity increases in humans from about six to eight weeks of gestation and raises during the late gestational period (27-32 weeks). It remains high for about one month after birth and then begins to decline. Adults have less than 10% the lactase activity present in infants and adult levels are reached in most of the world’s population by the age of five to seven years. However, in people of western Northern European ancestry and milk-dependent Nomadic tribes of Saharan Africa the levels of lactase remain at, or slightly below, infant levels throughout adulthood.
The production of maltose, maltotriose, and limit dextrins by pancreatic α-amylase occurs in the duodenum. Sucrase-isomaltase activity and β-glycosidase activity are highest in the jejunum. Glucoamylase activity increases progressively along the length of the small intestine, and is highest in the ileum.
Some starches, particularly those high in amylose or less well hydrated (e.g., starch in dried beans), are not well digested and enter the colon where colonic bacteria metabolize them, producing gases, short-chain fatty acids, and lactate. The major short chain fatty acids are acetic acid ( two carbons), propionic acid (three carbons) and butyric acid (four carbons). They are absorbed by the colonic mucosal cells where they can provide a substantial energy source. Some of these fatty acids may travel to the liver through the hepatic portal vein.
The hexoses, glucose, galactose and fructose, are polar molecules that require transporter proteins for entry into and exit from cells. Two distinct groups of hexose transporters have been identified and classified based on their dependence on cellular energy: GLUTs, facilitative GLUcose Transporters, transport hexoses down a concentration gradient and do not require an input of energy. SGLUT1, a Sodium GLUcose co-Transporter located at the apical side of the enterocyte and on absorptive cells of the kidney, and SGLUT2, located on the absorptive cells of the kidney, transport glucose and galactose against a concentration gradient.
Major Hexose Transporters | |
SGLUT1 SGLUT2 |
Apical surface of intestinal enterocytes (GSGLUT1), kidney tubules (SGLUT1 and SGLUT2); Cotransports one molecules of glucose or galactose from the intestinal lumen along with two sodium ions; Does not transport fructose |
GLUT1 | The primary glucose transporter in the vascular enthelia and glial cells of the brain and erythrocyte; Expressed in cell types with barrier functions: blood-brain barrier, blood-retinal barrier, blood-placental barrier, blood-testis barrier; Km = 1mM (high affinity for glucose); Does not transport fructose |
GLUT2 | Liver, pancreatic β cells, small intestine, and kidney; Transports glucose, galactose, and fructose; Km ~ 15 - 20 mM (low affinity for glucose); High Vmax (high capacity for glucose transport); Serves as a “glucose sensor” in pancreatic β cells; The major glucose transporter releasing glucose from intestinal enterocytes into the portal circulation |
GLUT3 | Brain, placenta, and testis; Transports glucose and galactose, not fructose; The primary glucose transporter in neurons; Km = 1mM (high affinity for glucose) |
GLUT4 | Skeletal muscle, cardiac muscle, adiposytes; insulin causes an increase in the number of GLUT4 molecules in the plasma membranes of muscle cells and adipocytes by mobilizing GLUT4-containing cytosolic membranes to the cell surface; Km = ~5mM (moderately high affinity for glucose) |
GLUT5 | Small intestine, sperm, brain, kidney, adipocytes, and muscle cells; Transports fructose, not glucose or galactose |
Click The Image
SGLUT IN INTESTINE & KIDNEY
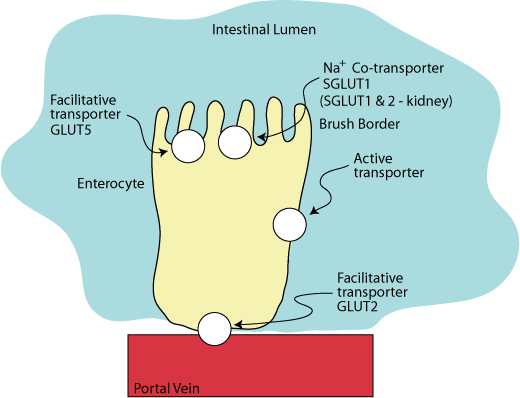
SGLUT 1 on the apical surface of enterocytes in the intestine and SGLUT 1 & 2 in the kidney proximal tubule are hexose-sodium co-transporters. In the intestinal enterocytes GLUT5, a facilitative fructose transporter, is also located on the apical surface and GLUT2, which transports glucose, glalctose and fructose, is located on the basal surface.
SODIUM CO-TRANSPORT
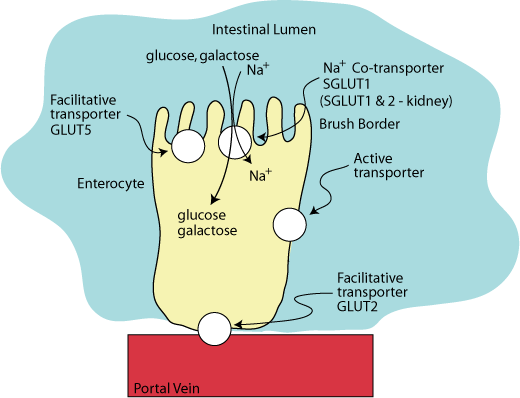
Two sodium ions are transported with each molecule of glucose or galactose into the enterocyte from the intestinal lumen against a concentration gradient.
SODIUM-POTASSIUM ATPASE
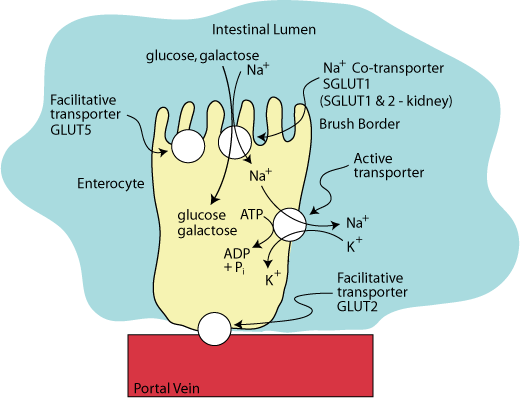
The driving force for this transport against a concentration gradient is provided by the hydrolysis of ATP by an active transporter called the “SodiumPpotassium ATPase”, which is located in the basal-lateral surface of the enterocyte. It transports three sodium ions from the inside of the enterocyte in exchange for two potassium ions.
FACILITATIVE TRANSPORT
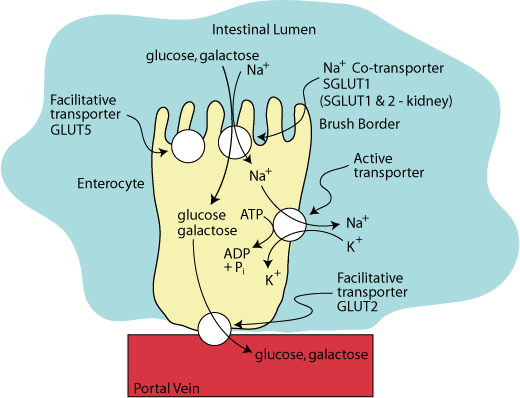
Glucose and galactose leave the enterocyte though GLUT2, a facilitative transporter located on the basal surface of the cell, from which they enter the blood via the portal vein.
FRUCTOSE FACILITATIVE TRANSPORT
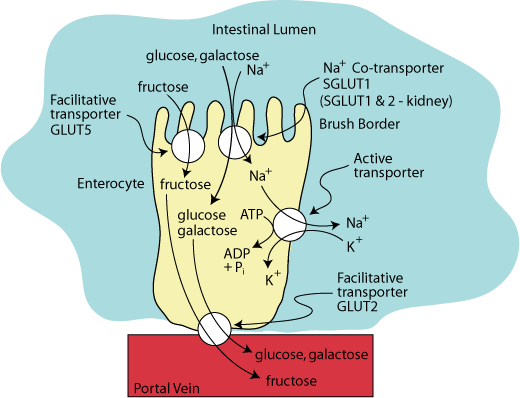
Fructose enters the enterocyte by GLUT5, a facilitative transporter on the apical surface of the enterocyte, and exits the enterocyte by the same GLUT2 facilitative transporter that transports glucose and galactose out of the enterocyte into the blood.
SGLUT2 IS A DRUG TARGET
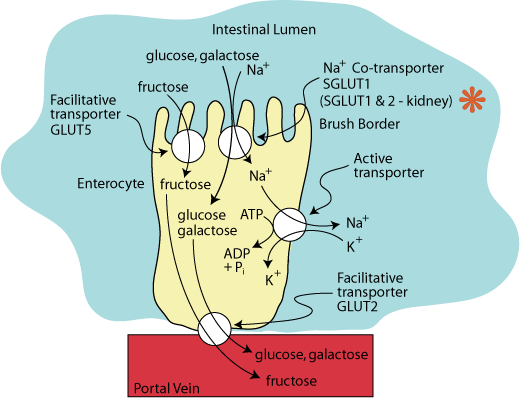
SGLUT2 is the target of drugs currently in clinical trials for the treatment of type 2 diabetes (Dapagliflozin, Remogliflozin, Sergliflozin). They inhibit SGLUT2 transport activity, thereby preventing the re-absorption of glucose (and galactose) from the kidney tubule for the purpose of lowering the concentration of glucose in the blood.
SGLUT 2 (SGLT2) REABSORBS 90% Of GLUCOSE FILTERED BY THE KIDNEY
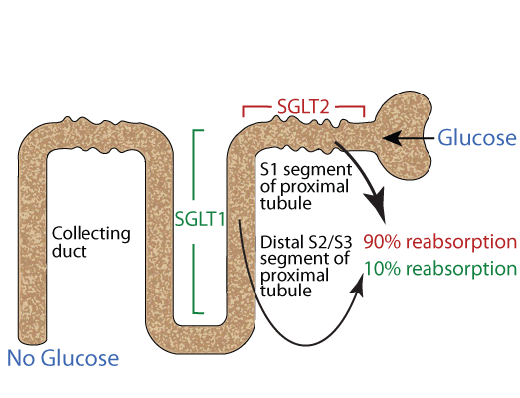
SGLUT 2 (SGLT 2) is located in the S1 segment of the proximal tubule of the nephron of the kidney where it readsorbes approximately 90% of the glucose filtered by the golmerulus. The remaining approximately 10% of the filtered glucose is reabsorbed by SGLUT 1 (SGLT 1).
CANAGLIFLOZIN, THE FIRST FDA APPROVED
SGLUT 2 (SGLT 2) INHIBITOR
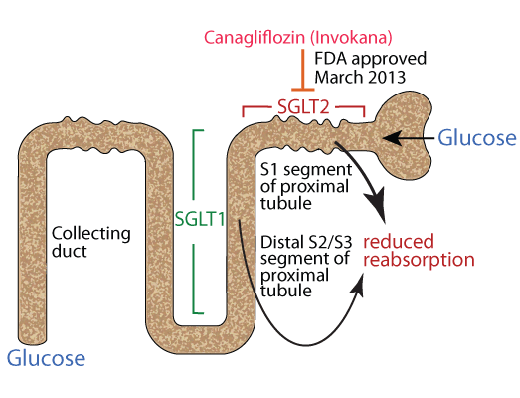
In March 2013 the FDA approved Canagliflozin (Invokana), a specific inhibitor of SGLUT 2 (SGLT 2) for the treatment of diabetic hyperglycemia. A 300 mg/day dose of Canagliflozin reduces the renal threshold for glucose from approximately 170-200 mg/dL to approximately 70-90 mg/dL.
GLUTS are integral membrane glycoproteins (sugar molecules covalently attached) with 12 membrane-spanning domains that transport hexoses between cells and the blood. SGLUT1 and SGLUT2 are not members of the GLUT family. Several GLUT isoforms, all perform a similar function, the transport of glucose in and out of cells, but different GLUTS have different Kms and Vmaxs and are expressed in different cell types commensurate with different cell-type-specific uses of glucose. For example, GLUT2, which has a high Km (low affinity for glucose) and a high Vmax (high capacity for glucose transport), is the major GLUT in the liver and pancreatic β cells.
The liver does not depend on glucose as an energy source, but rather, uses glucose mainly as a source of carbons for the synthesis of other molecules, most notably the energy storage molecules triacylglycerol (fats) and glycogen, when blood glucose is abundant from the diet soon after a meal. When dietary glucose is scarce, several hours after a meal, the liver does not use carbon from glucose to synthesize triacylglycerols or glycogen, as this would deplete blood glucose and deprive other glucose-dependent tissues of their essential energy source. Rather, the liver hydrolyzes its glycogen stores and synthesizes glucose from non-carbohydrate sources for export to maintain blood glucose homeostasis (at ~ 5mM). GLUT2, with its high Km and a high Vmax, is well suited to the liver’s use of glucose. Its high Km limits glucose entry into the liver when blood glucose is low. Its high Vmax allows rapid entry of glucose into the liver when blood glucose is high. In contrast, the brain is a glucose-dependent tissue and its neurons have GLUT3 with a low Km (high affinity for glucose), which ensures that the brain receives the glucose it requires for its survival, even when blood glucose concentration declines below normal.
Click The Image
AT NORMAL OR LOW GLUCOSE GLUT4 RESIDES IN CYTOSOLIC SEQUESTRATION VESSICLES
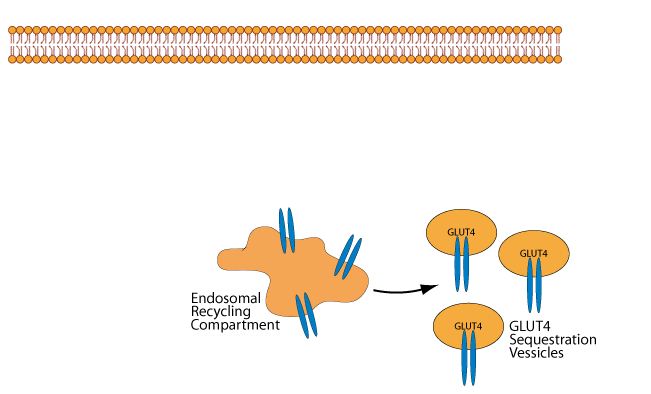
At normal or low blood glucose concentration, GLUT4 in skeletal muscle cells and adipocytes is located primarily in cytosolic vessicles called “GLUT4 Sequestration Vessicles” and in the endosomal recycling compartment, which can give rise to them.
INSULIN SIGNALING INDUCES GLUT 4 TO BECOME ASSOCIATED WITH THE PLASMA MEMBRANE
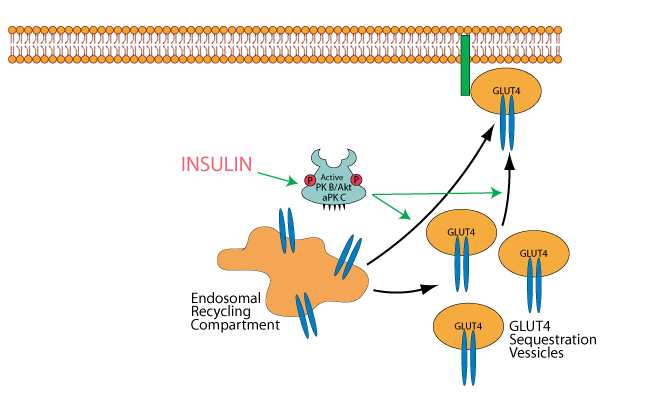
Insulin, acting through its activation of protein kinaseB/AKT [PKB/AKT] and atypical protein kinase C [aPKC] causes the mobilization of GLUT4 Sequestration Vessicles and possibly also the endosomal recycling compartment to the plasma membrane in skeletal muscle cells and adipocytes.
INSULIN PROMOTES THE INSERTION OF GLUT 4 INTO THE PLASMA MEMBRANE
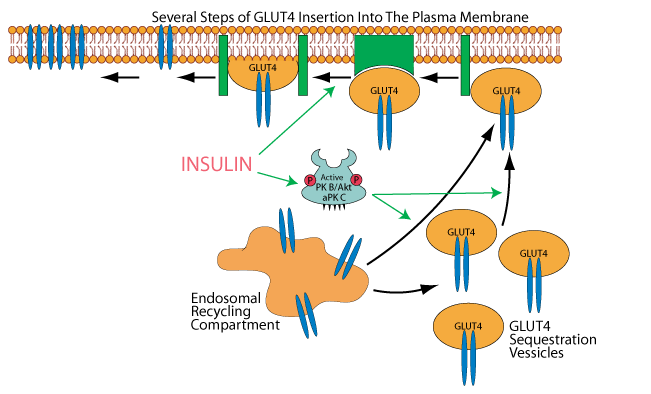
GLUT 4 is then inserted into the plasma membrane in several steps, one of which is believed to be promoted by insulin signaling, but how insulin signaling accomjplishes this is not elucidated.
INSULIN INHIBITS THE REMOVAL OF GLUT 4 BY ENDOCYTOSIS FROM THE PLASMA MEMBRANE
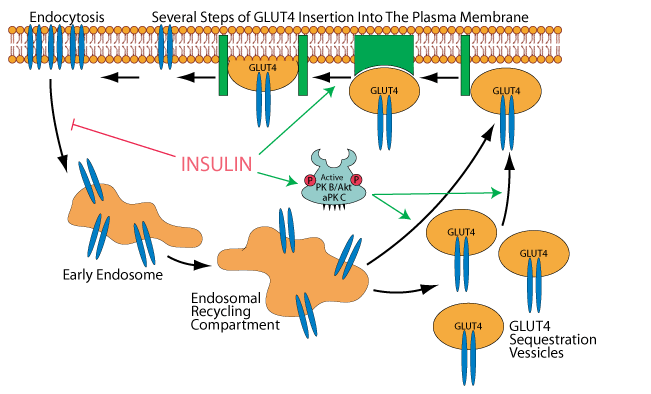
Insulin signaling also inhibits endocytosis of GLUT 4, thereby causing an increase in its concentration in the plasma membrane. Clearance of glucose from the bood by its GLUT 4 transport into muscle and adipose tissue is a major mechanism for maintaining blood glucose homeostasis following glucose ingestion during a meal.
EXERCISE AND METFORMIN INDUCE THE MOBILIZATION OF GLUT 4 TO THE PLASMA MEMBRANE
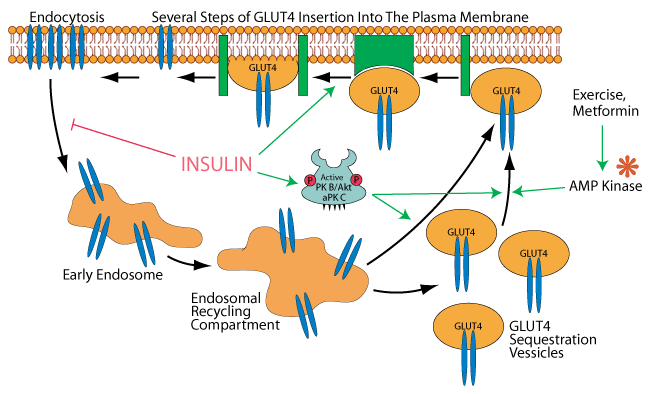
Current evidence inticates that AMP kinase [AMPK], which is activated when AMP accumulates, for example, in skeletal muscel cells during exercise, can induce the mobilization of GLUT4 to the plasma membrane. Metformin, a drug used to treat type 2 diabetes activates AMPK.
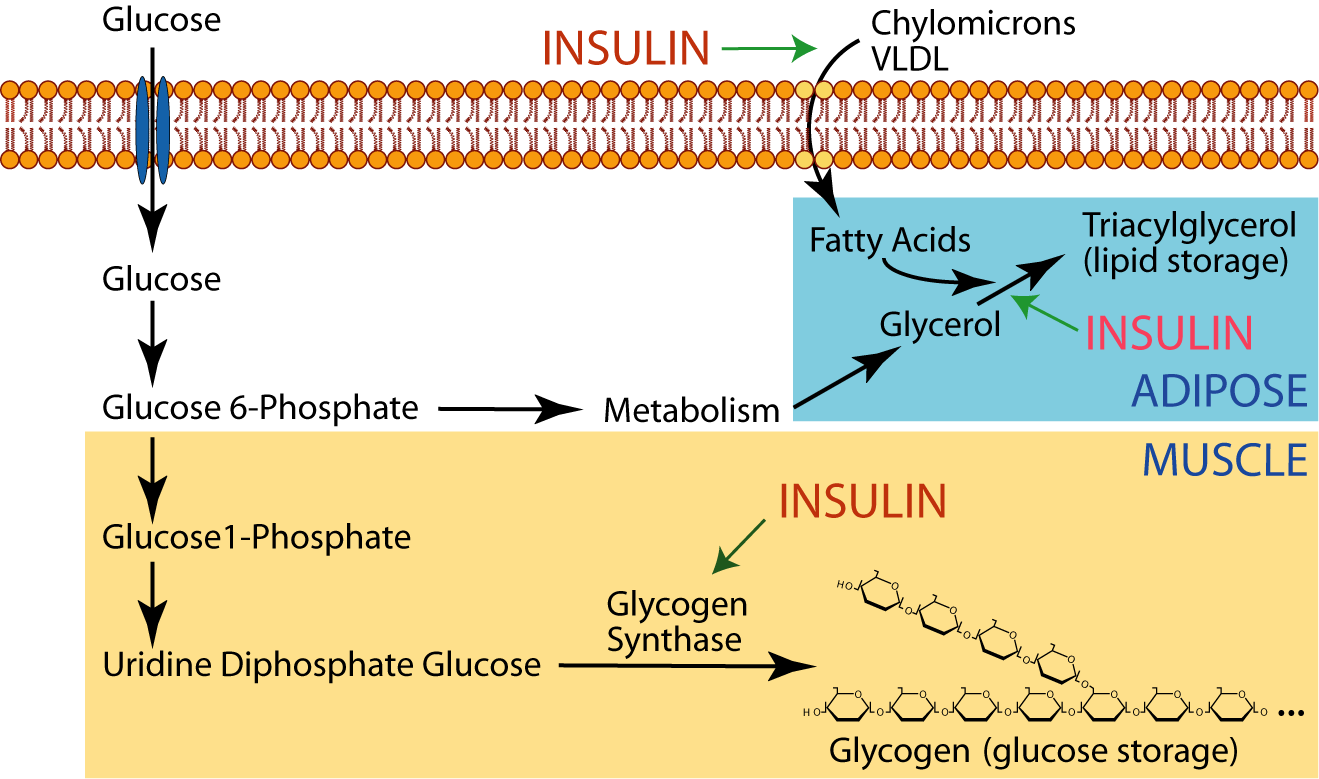
Adipose tissue uses the excess glucose for the production of glycerol, which it esterifies with fatty acids delivered to it by lipoproteins in the blood to synthesize triacylglycerol (lipid) for long-term storage. Insulin promotes triacylglycerol synthesis.