METABOLIC ENERGY
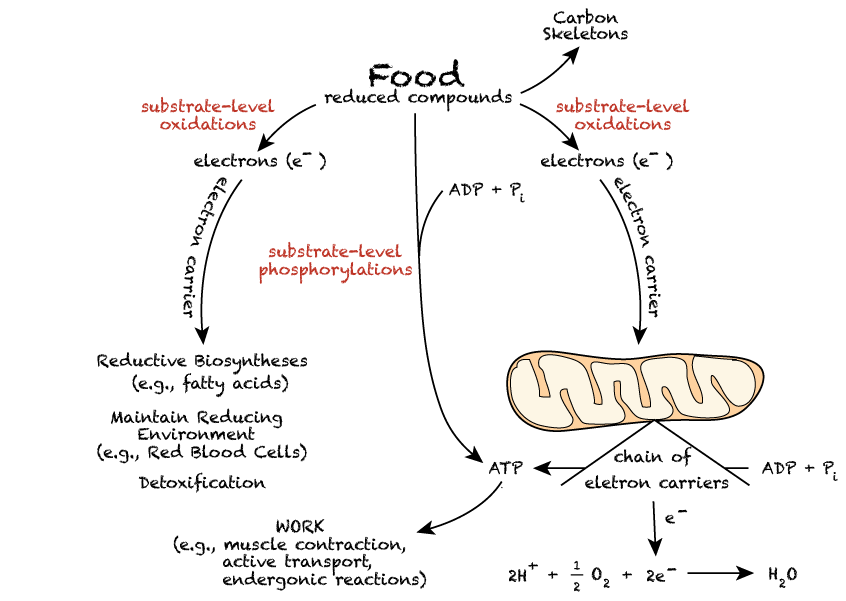
Food molecules provide energy because they are reduced compounds, sources of electrons that are released by oxidative biochemical reactions. Enzymes that catalyze biochemical oxidations use small-molecule electron acceptors (electron carriers) as cofactors that capture electrons and donate them to various biochemical reductive reactions. One such cofactor, nicotinamide adenine dinucleotide (NAD+) accepts electrons and donates them predominantly for the production of ATP energy. A related cofactor, nicotinamide adenine dinucleotide phosphate (NADP+) accepts electrons and donates them predominantly for reductive biosynthetic reactions in which the reaction products are more reduced than the substrates, or to maintain a reducing environment inside cells. NADP+ does not donate electrons for the production of ATP energy.
Oxidations that do not occur as part of the electron transport chain (in mitochondria) are referred to as "substrate-level" oxidations. Likewise, the production of ATP by processes that do not involve the participation of the electron transport chain are referred to as "substrate-level" phosphorylations.
Food molecules, in addition to serving as a source of electrons, also provide carbon skeletons for the synthesis of other biological molecules.
SMALL MOLECULE CO-FACTORS REQUIRED FOR METABOLISM
All living organisms require a continual input of energy to maintain the multitude of metabolic reactions in a state far from equilibrium (equilibrium = death).Three major purposes for energy in biological organisms:
- Mechanical Work - e.g., muscle contraction
- Active Transport - to maintain chemical balance, e.g., cells need to continually pump out Na+ ions which are taken in as part of transport mechanisms for other molecules - achieved by the Na+/K+ ATPase, which uses energy to pump Na+ against a concentration gradient. Active transport accounts for about 10% - 30% of the energy needs of an organism.
- Synthesis of essential biological molecules (e.g., protein synthesis).
Adenosine Triphosphate [ATP] is the universal currency of energy in biological processes within cells. It is the link between energy-producing and energy-utilizing systems. Its hydrolysis yields useful energy.
Structural basis for the phosphoryl transfer potential of ATP
- electrostatic repulsion between proximal negative charges
- ADP and Pi have a larger number of resonance forms than ATP
The energy liberated in the hydrolysis of ATP can be harnessed to drive reactions that require an energy input.
- Reactions that wouldn't proceed spontaneously, because they required an input of energy, can proceed if they are coupled to ATP hydrolysis.
- ATP hydrolysis shifts the equilibria of coupled reactions. The equilibrium of a reaction is changed by 108 per ATP molecule hydrolyzed. Thus, if 3 molecules of ATP are hydrolysed in a coupled reaction, the equilibrium of the reaction would be changed by 1024.
ATP is formed from ADP and Pi when fuel molecules are oxidized by chemotrophs (or when light is trapped by phototrophs)
This ATP-ADP cycle is the fundamental mode of energy exchange in biological systems.
- ATP turnover is high. The half-life of an ATP molecule in many tissues is approximately one minute.
- A resting human uses approximately 40 kg of ATP in 24 hours = approximately 1000 tons in a lifetime.
- During strenuous exertion (e.g., sprinting) ATP use can be a high as 0.5 kg / min.
NADH and FADH2 are the major electron carriers in the oxidation (dehydrogenation) of fuel molecules.
In aerobic organisms the ultimate electron acceptor is O2. However, electrons are not transferred directly from fuel molecules and their breakdown products to O2. They are first transferred to the electron carriers (substrate-level oxidations). The reduced forms of these carriers then transfer their high-potential electrons to O2 by means of an electron transport chain located in the inner membrane of the mitochondria.
- Nicotinamide adenine dinucleotide [NAD+] structure; in NAD+, R = H, in NADP+ R = PO32-
- In the oxidation (dehydrogenation) of a substrate, the nicotinamide ring of NAD+ accepts a hydrogen ion and two electrons - the equivalent of a hydride ion.
- The reduced form of this electron carrier is called NADH. In the oxidized form, the pyridine ring nitrogen is tetravalent and carries a positive charge. In the reduced form, NADH, the nitrogen atom is trivalent.
- Reaction type that uses NAD+ (typically, but not exclusively, oxidation of an alcohol to a carbonyl).
- Flavin adenine dinucleotide [FAD]
- The reactive part of FAD is its isoalloxazine ring. It accepts two electrons, and two protons.
- Reaction type that uses FAD (generally, but not exclusively, a dehydrogenation of a saturated compound with the formation of a double bond)
- NADPH is the major electron donor in reductive biosynthesis.
- In most biosynthetic reactions the precursors are more oxidized than the products so reducing power is required. e.g., in fatty acid biosynthesis a keto group is sequentially reduced via an alcohol and an unsaturated aliphatic chain.
- NADPH differs from NADH in that the 2'-OH of its adenosine moiety is esterified with phosphate. This extra phosphate is a tag that directs this reducing agent to discerning biosynthetic enzymes.
- NADPH carries electrons in the same way as NADH.
- NADPH is used almost exclusively for reductive biosynthesis, whereas NADH is used primarily for the generation of ATP.
Why have both NADP+ and NAD+ ?- The cellular ratio of NADP+/NADPH = 0.014
- The cellular ratio of NAD+/NADH = 700
- The cell can thus maintain a high level of reducing equivalents for biosynthetic reactions while simultaneously maintaining high capacity for substrate-level oxidations so that energy can be generated.
- In most biosynthetic reactions the precursors are more oxidized than the products so reducing power is required. e.g., in fatty acid biosynthesis a keto group is sequentially reduced via an alcohol and an unsaturated aliphatic chain.
- The reactive part of FAD is its isoalloxazine ring. It accepts two electrons, and two protons.
Coenzyme A is a universal carrier and donor of acyl groups (originally "A" stood for acetyl because Coenzyme A was discovered as acetyl Coenzyme A, but it was subsequently recognized that many different acyl groups were attached to the Coenzyme).
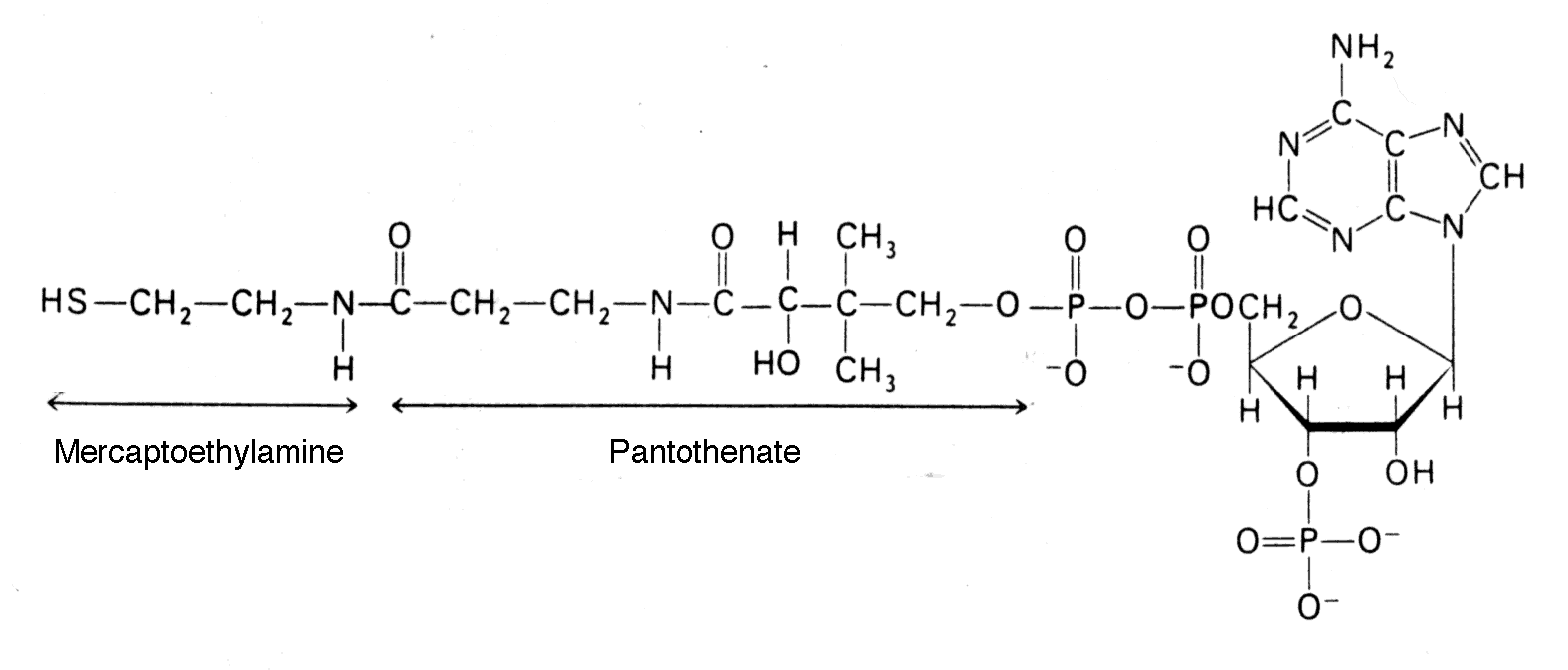
- The terminal sulfhydryl group is the reactive site, which forms thioesters with acyl (not only acetyl) groups.
- Acetyl CoA is a common intermediate in different catabolic pathways (e.g., glycolysis, beta-oxidation of fatty acids and amino acid degradation all supply the cellular pool of acetyl CoA).