THE POSTABSORPTIVE STATE AND THE ACIDOTIC STATE:
EXAMPLES OF AMINO ACID FLUX IN THE BODY
The fasting state and the acidotic state provide examples of the inter-organ flux of amino acids necessary to maintain the free amino acid pool in the blood and supply tissues with their required amino acids, and to maintain physiological pH. During an overnight fast, protein synthesis in the liver and other tissues continues, but at a diminished rate compared to the postprandial state (after eating). Net degradation of labile protein occurs in skeletal muscle, which contains the body’s largest protein mass, and in other tissues. The net degradation of protein affects functional proteins, such as skeletal muscle myosin, which are sacrificed to meet more urgent demands for amino acids in other tissues, and to provide carbon skeletons for gluconeogenesis by the liver to meet the needs for glucose, particularly of brain and red blood cells.
The pattern of inter-organ flux of amino acids is affected by conditions that change the supply of fuels (for example the overnight fast, a mixed meal, a high protein meal), and by conditions that increase the demand for amino acids (metabolic acidosis, surgical stress, traumatic injury, burns, wound healing, and sepsis). The flux of amino acid carbon and nitrogen in these different conditions is dictated by several factors:
- Ammonia (NH4+) is toxic. Consequently, it is transported between tissues as alanine or glutamine. Alanine is the principal carrier of amino acid nitrogen from other tissues back to the liver, where the nitrogen is converted to urea and subsequently excreted into the urine by the kidneys. The amount of urea synthesized is proportional to the amount of amino acid carbon that is oxidized as fuel.
- The pool of glutamine in the blood serves several essential metabolic functions. It provides ammonia for excretion of protons in the urine as NH4+. It serves as a fuel for the gut, the kidney, and the cells of the immune system. Glutamine is also required by the cells of the immune system and other rapidly dividing cells in which its amide group serves as the source of nitrogen for biosynthetic reactions; glutamine is a major donor of nitrogen for biosynthetic reactions. In the brain, the formation of glutamine from glutamate and NH4+ provides a means of removing ammonia and of transporting glutamate between cells in the brain. The utilization of blood glutamine is prioritized. During metabolic acidosis the kidney becomes the predominant site of glutamine uptake, at the expense of glutamine utilization in other tissues. On the other hand, during sepsis, cells involved in the immune response (macrophages, hepatocytes) become the preferential sites of glutamine uptake.
- The branched chain amino acids — valine, leucine, isoleucine — form a significant portion of the average protein, and can be converted to TCA cycle intermediates and utilized as fuels by almost all tissues. They are also the major precursors of glutamine. Except for the branched chain amino acids and alanine, aspartate, and glutamine, the catabolism of amino acids occurs principally in the liver.
- Amino acids are major gluconeogenic substrates, and most of the energy obtained from their oxidation is derived from oxidation of the glucose formed from their carbon skeletons. A much smaller percentage of amino acid carbon is converted to acetyl CoA or to ketone bodies and oxidized. The utilization of amino acids for glucose synthesis for the brain and other glucose-requiring tissues is subject to the hormonal regulatory mechanisms of glucose homeostasis.
- The relative rates of protein synthesis and degradation — protein turnover — determine the size of the free amino acid pools available for the synthesis of new proteins and for other essential functions. For example, the synthesis of new proteins to mount an immune response is supported by the net degradation of the proteins in the body.
The release of amino acids from skeletal muscle is stimulated during an overnight fast by the decrease of insulin and increase of glucocorticoid levels in the blood. Insulin promotes uptake of amino acids and the general synthesis of proteins. The fall in blood insulin during an overnight fast results in net proteolysis and release of amino acids, because the equilibrium between protein synthesis and protein degradation is shifted toward degradation.
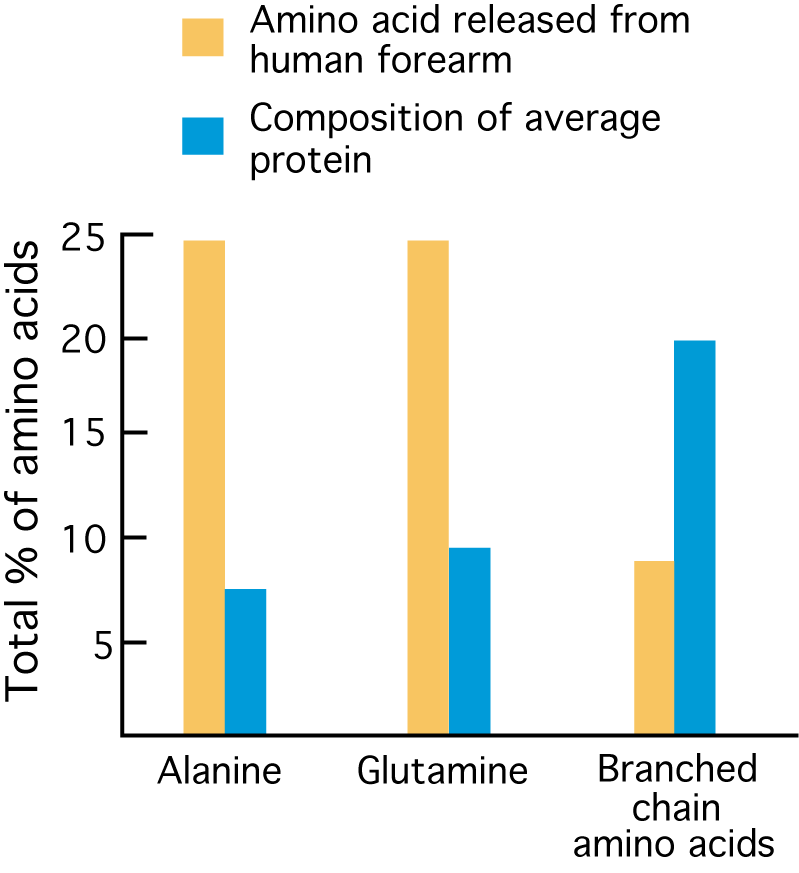
- Because of its large mass, skeletal muscle is a major site of protein synthesis and breakdown.
- Efflux of amino acids from skeletal muscle supports the amino acid pool in the blood.
- Alanine and glutamine account for approximately 50% of all amino acids released from skeletal muscle.
- The graph at the right shows the difference between alanine, glutamine, and branched chain amino acids in venus blood leaving the human forearm compared to their percentage representation in average skeletal muscle protein composition. Alanine and glutamine represent a much higher percentage of total nitrogen released than originally present in the degraded proteins, evidence that they are synthesized in the muscle. The branched chain amino acids are released in a much lower percentage than their representation in the degraded protein, evidence that they are catabolized in muscle.
- The branched chain amino acids, aspartate, and glutamate supply the amino groups for alanine and glutamine production.
- Alanine is a major transporter of ammonia (a neurotoxin) from extra-hepatic tissues to the liver (see the “Alanine Cycle” below) for disposal as urea. Its carbon skeleton is used for energy production by the liver or for glucose synthesis in response to hypoglycemia. Alanine is produced by transamintion of pyruvate, a relatively abundant α-ketoacid.
- A major fate of branched-chain amino acids is to provide carbon skeletons for glutamine formation.
- oxidation of the branched chain amino acids (valine, leucine, isoleucine) to produce energy, first by removal of the α-amino nitrogen by transamination and then by supplying the carbon skeletons to the TCA cycle; glucose is spared as a result of the use of this alternate energy source during fasting; α-ketoglutarate produced by the TCA cycle is used in the synthesis of glutamine for export
- entry of carbon skeletons to the TCA cycle at succinyl CoA and acetyl CoA
- exit of carbon skeletons from the TCA cycle as α-ketoglutarate
- transamination of α-ketoglutarate to glutamate or glutamate formation from α-ketoglutarate and ammonia via glutamate dehydrogenase
- glutamine production by addition of the amide nitrogen to glutamate (glutamine synthetase)
- Chronic stresses induce the breakdown of tissue protein, — mainly in muscle — leading to the increase in the level of amino acids. Most amino acids are metabolized in the liver, but the branched chain amino acids are metabolized mainly in extra-hepatic tissues, where the carbon skeletons of isoleucine and valine are largely converted to glutamine, which is a nitrogen donor for the synthesis of many biological molecules, or is excreted by the kidney.
- Depending on the particular stress, different tissues have different needs for glutamine or its amide nitrogen. For example, serious infections cause a proliferation of cells of the immune system to counteract the infections agent, requiring increased immune cell division and cell growth. Nitrogen for the synthesis of several biological molecules, such as ribonucleotides and deoxyribonucleotides is donated by glutamine, and, therefore, the immune cells get most of the glutamine.
- In acidosis (and other stresses) cortisol signaling causes an increase of glutamine synthetase and branched-chain α-ketoacid dehydrogenase (see “Synthesis & Degradation > Branched-Chain AA” in the top menu) in muscle, leading to increased synthesis and export of glutamine, which the kidney uses as a source of ammonia to buffer excess protons in the urine, thereby promoting their excretion from the body.
Click The Image
Alanine Cycle: Production Of Extra-Hepatic Tissue Pyruvate
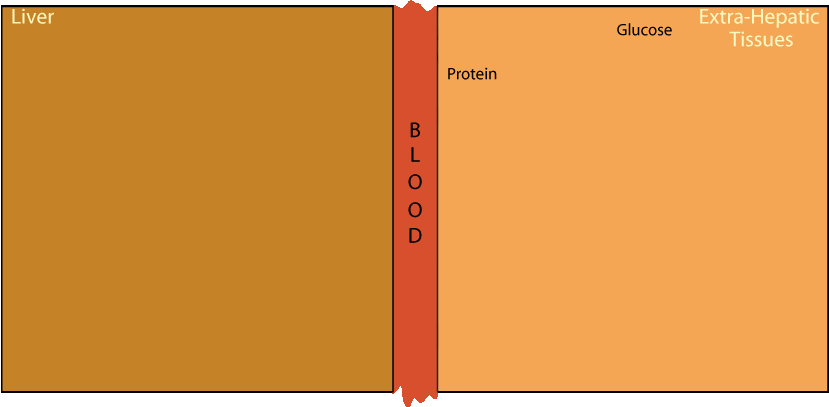
Pyruvate is a relatively abundant α-keto acid. It is produced mainly from glucose and, depending on the balance between protein synthesis and protein degradation, from protein degradation followed by amino acid degradation. Six of the 20 common amino acids degrade to yield pyruvate (see the diagram at the right below).
Alanine Cycle: Production Of Extra-Hepatic Tissue Pyruvate
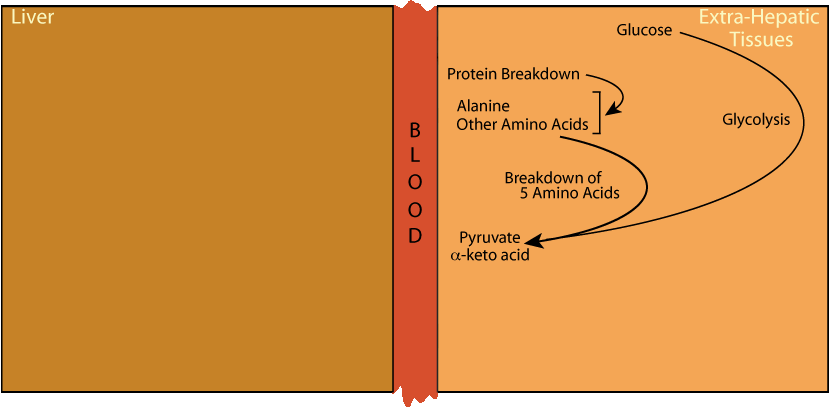
Glycolysis and the degradation of 5 amino acids produce pyruvate.
Alanine Cycle: Nitrogen For Production Of Extra-Hepatic Tissue Alanine
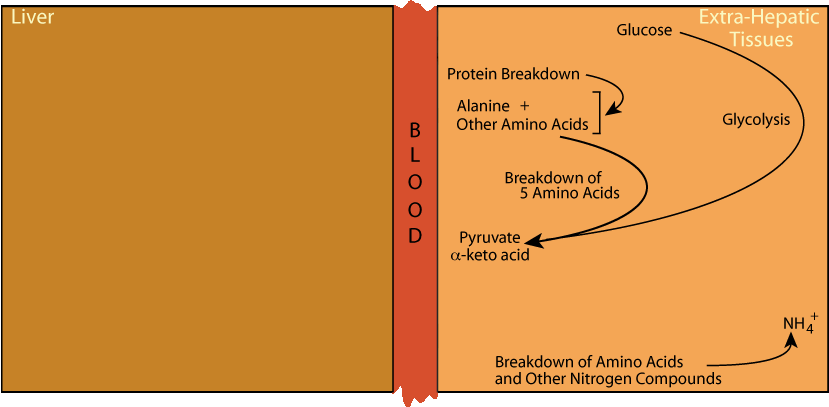
The pyruvate supplies the carbon skeletons for alanine production in extra-hepatic tissues. The NH4+ is supplied by the degdradation of various amino acids and other nitrogen-containing compounds.
Alanine Cycle: Glutamate Dehydrogenase & Transaminase
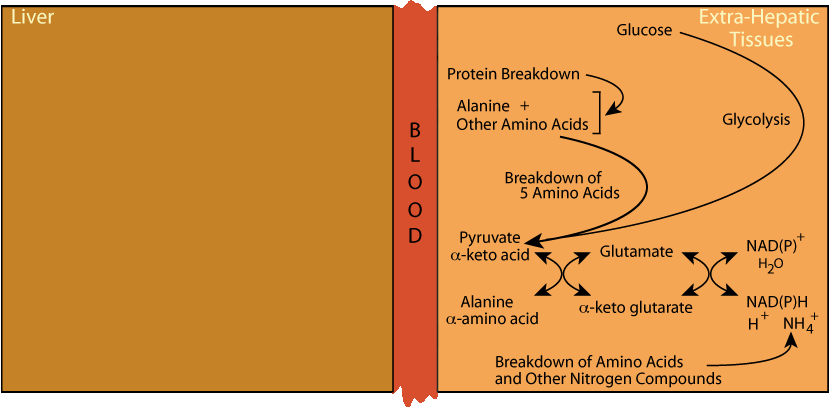
Glutamate dehydrogenase fixes the nitrogen from NH4+ to α-ketoglutarate to form glutamate, which donates it by transamination to pyruvate to form alanine.
Alanine Cycle: Alanine Transport To The Liver
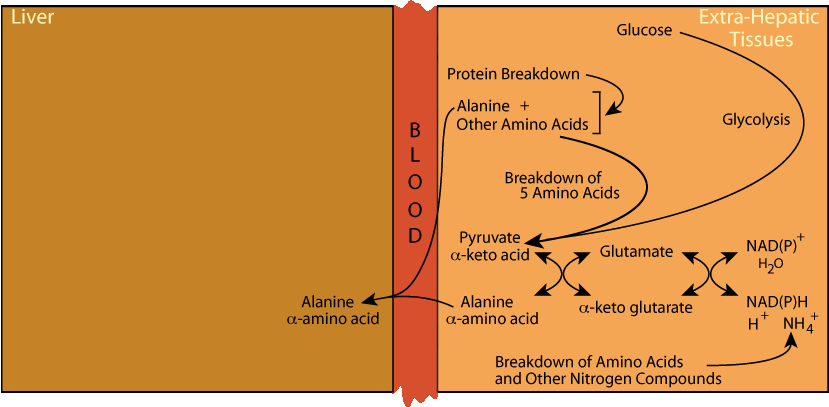
The resulting alanine, as well as that generated directly by protein breakdown, is transported to the liver,
Alanine Cycle: Transaminase & Glutamate Dehydrogenase
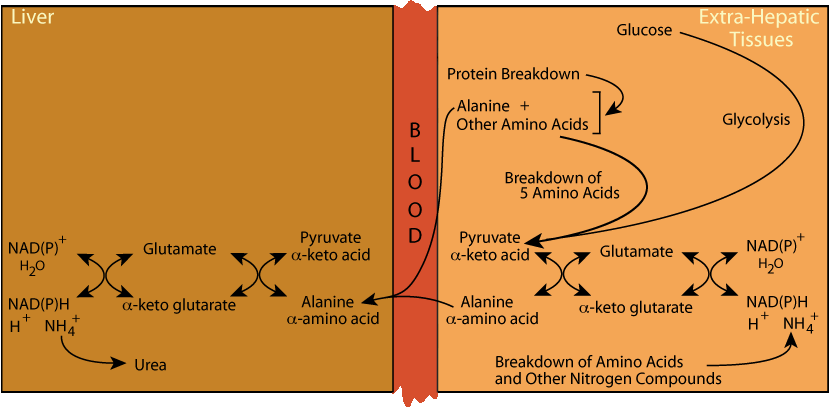
where transamination generates pyruvate and glutamate dehydrogenase removes NH4+ for disposal in urea.
Alanine Cycle: Energy From Pyruvate
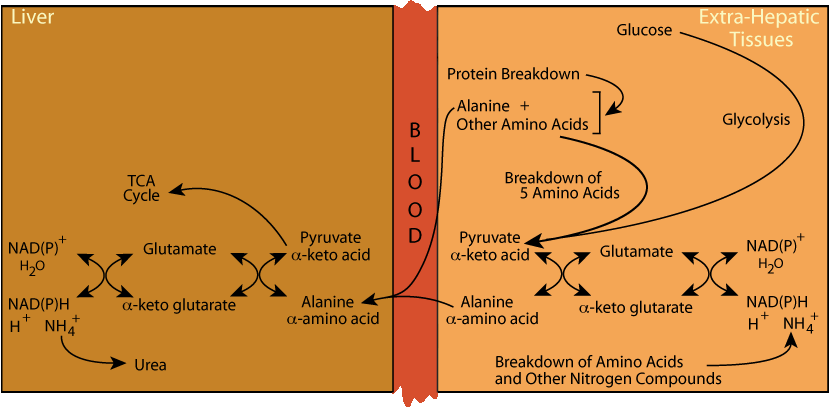
The pyruvate carbon skeleton can be used for the production of energy, or
Alanine Cycle: Glucose From Pyruvate
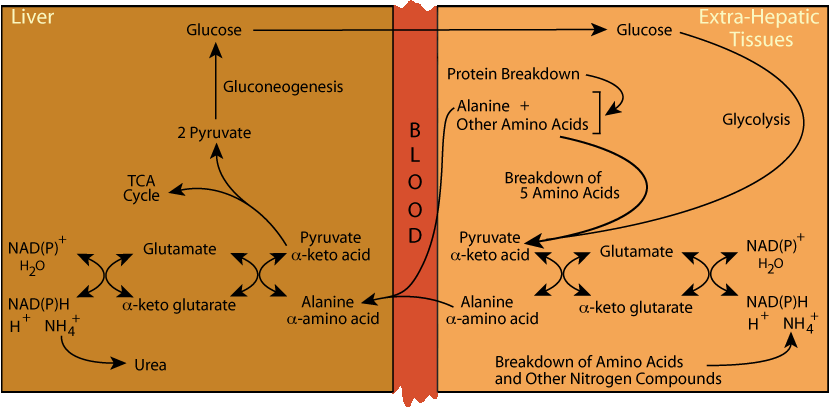
to complete the alanine cycle, during hypoglycemia the pyruvate carbon skeleton is used for the synthesis of glucose (gluconeogenesis), which the liver releases for energy production in extra-hepatic tissues, particularly those that depend on glucose as an energy source (e.g., brain, red blood cells, lens of the eye).
Hypoglycemia: Cortisol Signaling Provides Carbon Skeletons For Glucose Synthesis
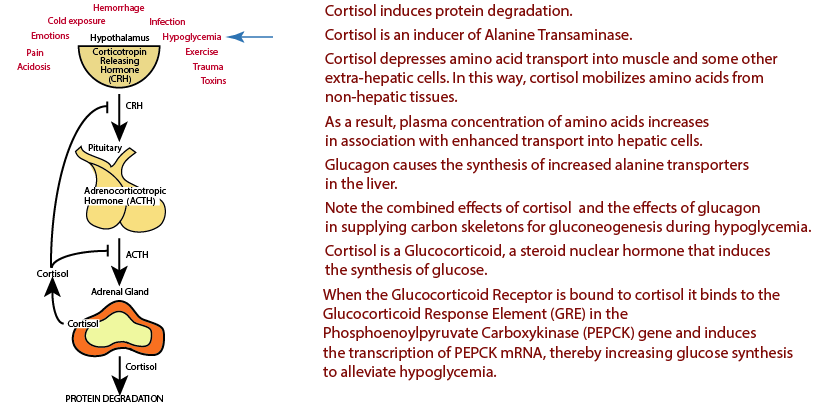
Hypoglycemia is one of the chronic stresses that induces the release of Cortisol via the Hypothalamic-Pitutary-Adrenal Axis (see “Introduction” in the top menu). Cortisol signaling via the Glucacorticoid Receptor induces protein degradation, particularly in muscle, to supply a source of carbon skeletons from amino acids for the production of glucose by the liver.
Click The Image
Amino Acid Degradation
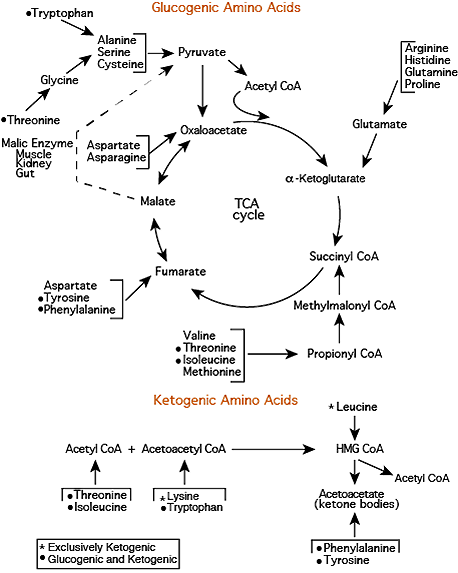
Carbon skeletons from 18 of the common 20 amino acids can be convereted either to pyruvate or to intermediates of the TCA cycle. Carbon skeletons from leucine and lysine are converted to precursors that are exclusively ketogenic and are not converted to either pyruvate or intermediates of the TCA cycle.
Amino Acid Degradation: Valine & Isoleucine
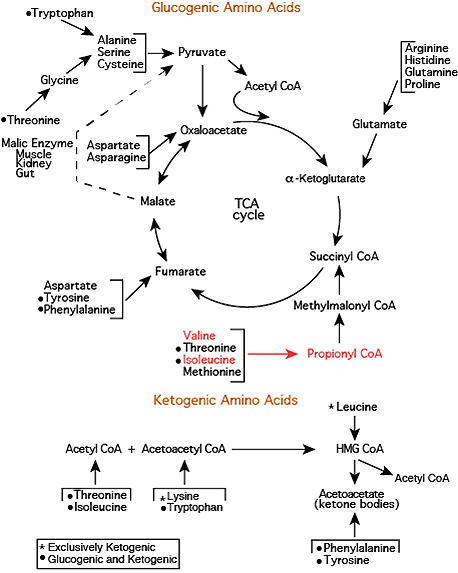
Branched chain amino acids — isoleucine, leucine, valine — are degraded predominantly in extra-hepatic tissues — mainly muscle — because they contain higher activities of the transaminases for the branched chain amino acids than does the liver, the tissue in which most amino acid degradation occurs. Isoleucine and Valine degrade to yield Propionyl CoA (see “Synthesis & Degradatioin > Branched-Chain AA” in the top menu).
Valine & Isoleucine Carbon Skeletons Enter The TCA Cycle
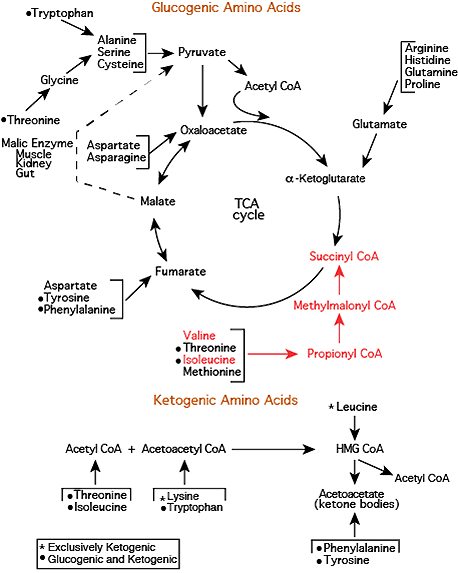
Propionyl CoA is converted to D-Methylmalonyl CoA by Propinyl CoA Carboxylase, D-Methylmalonyl CoA is converted to L-Methylmalonyl CoA by Methylmalonyl CoA Rascemase, and L-Methylmalonyl CoA is converted to Succinyl CoA, a TCA cycle intermediate, by Methylmalonyl CoA Mutase, one of only two reactions in humans that require vitamin B12.
TCA Cycle Intermediates From Valine & Isoleucine Carbon Skeletons
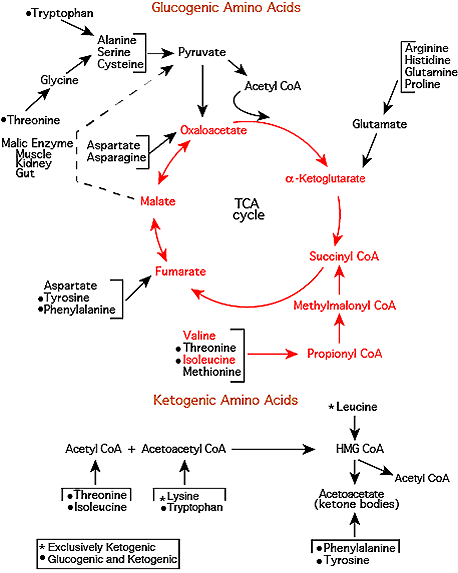
The Succinyl CoA carbon skeleton is converted to α-Ketoglutarate by TCA Cycle reactions.
Glutamate Dehydrogenase
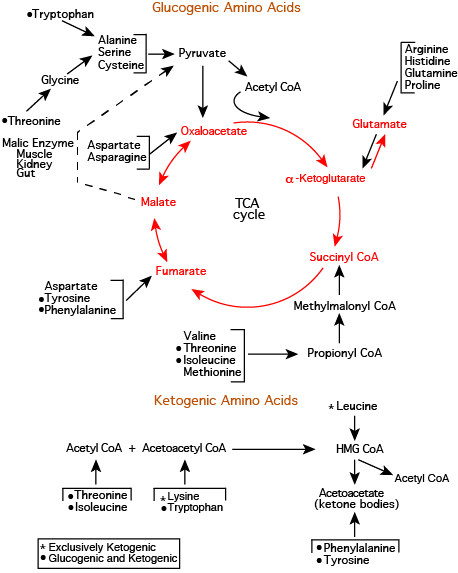
α-Ketoglutarate is drawn off by glutamate dehydrogenase, which converts it to Glutamate
Glutamine Synthetase
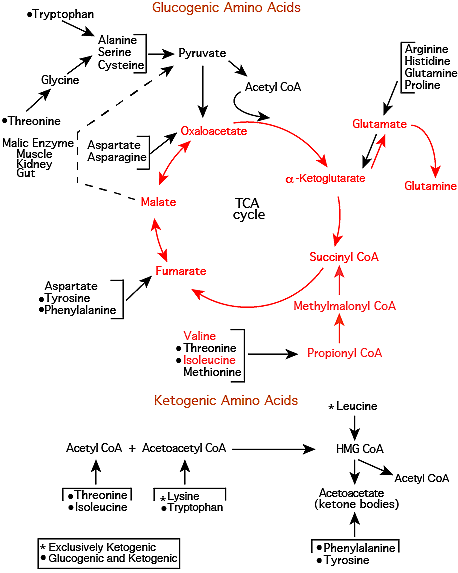
Glutamine synthetase converts the Glutamate to Glutamine, which is released into the blood.
Click The Image
Glutamine In The Kidney: Glutaminase
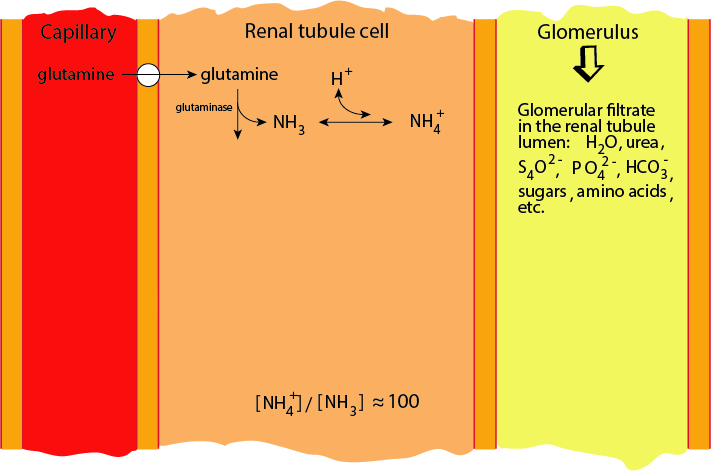
Glutamine is the source of ammonia to buffer excess protons for excretion in the urine. Glutaminase releases the amide nitrogen. In acidoses the activity of renal Glutaminase is increased.
Glutamate In The Kidney: Glutamate Dehydrogenase
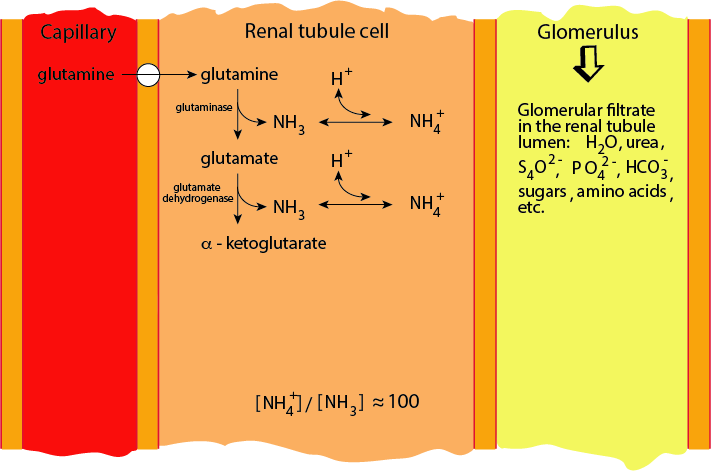
Glutamate Dehydrogenase releases the amino nitrogen. In acidosis renal Glutamate Dehydrogenase activity is increased.
Proton Elimination Into The Proximal Tubule Urine
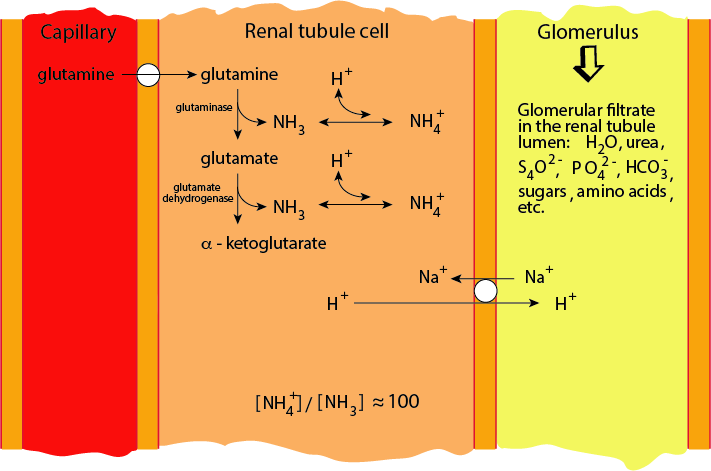
Excess protons are pumped across the renal tubule cell membrane into the lumen of the proximal tubule in exchange for sodium ions.
Ammonia Diffuses Across The Renal Tubule Plasma Membrane
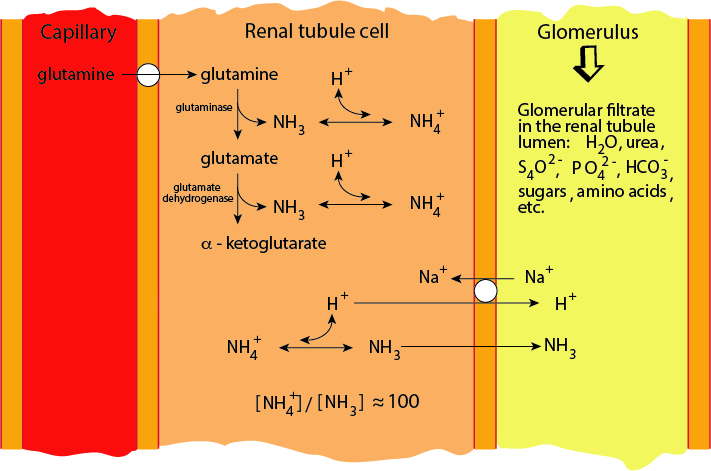
α-Ammonium ions derived from glutamine by Glutaminase and Glutamate Dehydrogenase are in equilibrium with ammonia. Ammonia, but not the ammoniuim ion diffuses across the renal tubule cell plasma membrane into the lumen of the proximal tubule.
Ammonia Buffers The Excess Protons
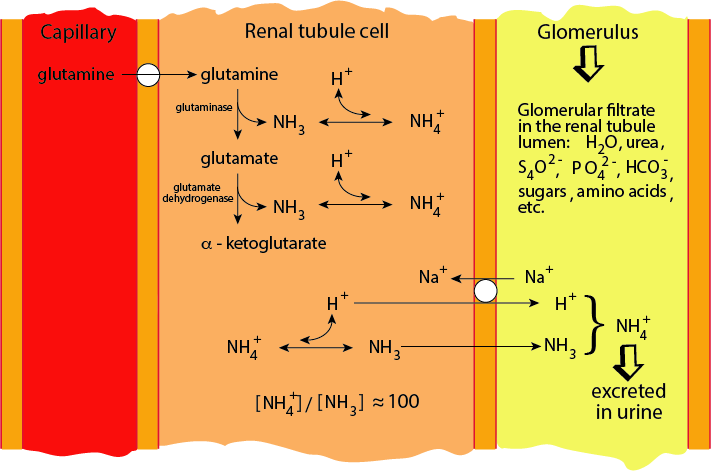
Excess protons in the proximal tubule urine combine with the ammonia and the resulting ammonium ion is excreted.
One of the primary roles of amino acid nitrogen is to provide ammonia in the kidney for the excretion of excess protons in the urine to alleviate acidosis. The rate of uptake of glutamine, the ammonia donor, from the blood and its utilization by the kidney depends mainly on the amount of acid that must be excreted to maintain a normal pH in the blood. During acidosis excretion of NH4+ increases several fold.
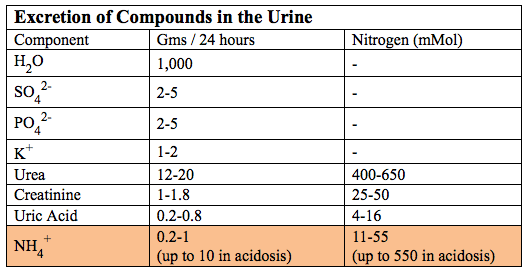
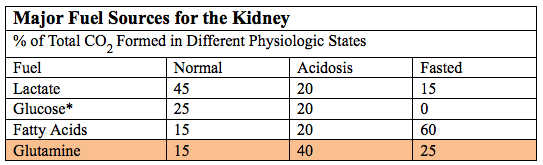
Note the difference in NH4+ excretion and the use of glutamine by the kidney between the normal state and acidosis
- ammonia increases proton excretion by providing a buffer for protons which are transported into the renal tubular fluid, and subsequently into the urine
- glutamine provides about two thirds of the NH4+ excreted
- uptake of glutamine by the kidney increases during metabolic acidosis to provide more NH3 to buffer excess protons for excretion in the urine as NH4+
- renal glutamine utilization for proton excretion takes precedence over the requirement of other tissues for glutamine
- glutaminase releases the amide nitrogen, glutamate dehydrogenase releases the α-amino nitrogen
- NH4+ does not cross the renal tubule cell membrane into the renal tubule because it is charged, but it is in equilibrium with NH3 ([NH4+]/[NH3] = ~100), which is uncharged and does cross the membrane
- In chronic metabolic acidosis the activities of renal glutaminase, glutamate dehydrogenase, phosphoenolpyruvate carboxykinase and mitochondrial glutamine transporter increase and correlate with increased urinary excretion of ammonium ions and increased renal gluconeogenesis from amino acids. Cortisol, released via the Hypothalamic-Pitutary-Adrenal Axis induces the liver to reduce glutaminase production in the periportal cells, thereby perserving glutamine for use by other tissues that respond to particular chronic stresses (e.g., the kidney in the case of acidosis).
Click The Image
Fate of α-Ketoglutarate In The Kidney
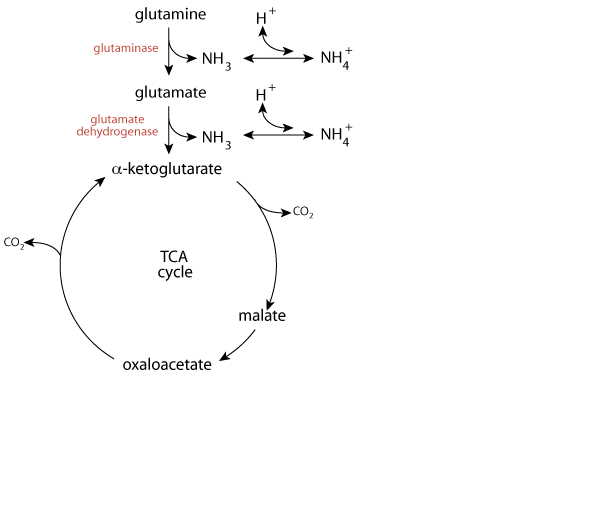
α-ketoglutarate resulting from the deamidation and deamination of glutamine enters the TCA cycle, where it loses a CO2 as it is converted to a four-carbon TCA cycle intermediate.
Fate of α-Ketoglutarate In The Kidney
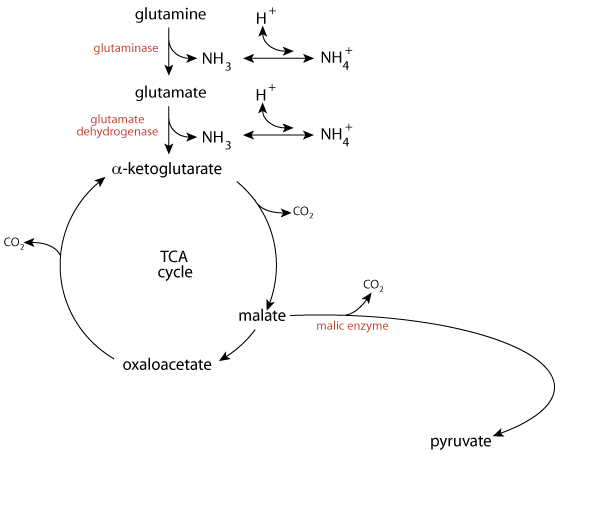
To be oxidized completely for the production of energy, it must first exit the TCA cycle, which it can do via mitochondrial malic enzyme (decarboxylating malate dehydrogenase).
Fate of α-Ketoglutarate In The Kidney
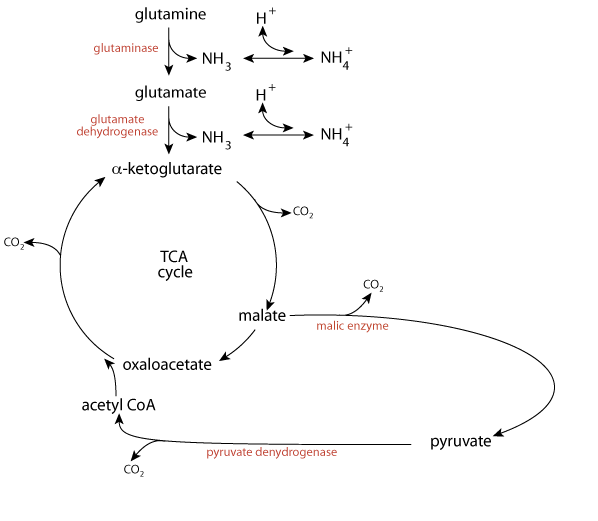
The resulting pyruvate can be converted to acetyl CoA (pyruvate dehydrogenase), which can enter the TCA cycle to be oxidized completely to CO2. Glutamine is normally used as a source of energy for the kidney, and even more so during fasting or acidosis.
Fate of α-Ketoglutarate In The Kidney
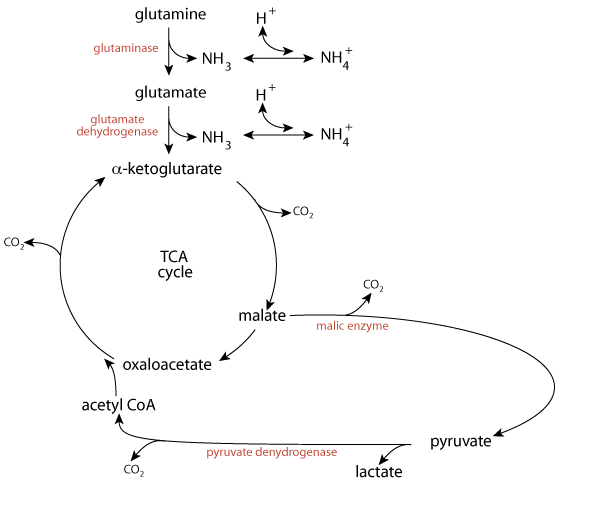
The pyruvate could be converted to lactate (lactate dehydrogenase) and returned to the blood, or
Fate of α-Ketoglutarate In The Kidney
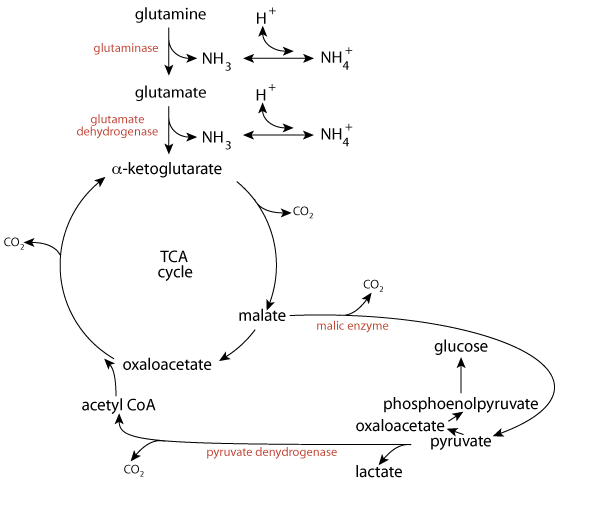
because, like the liver, the kidney is a glucogenic organ, the pyruvate could be converted to oxaloacetate (pyruvate carboxylase) and subsequently to phosphoenolpyruvate (phosphoenolpyruvate carboxykinase) and then to glucose.
Fate of α-Ketoglutarate In The Kidney
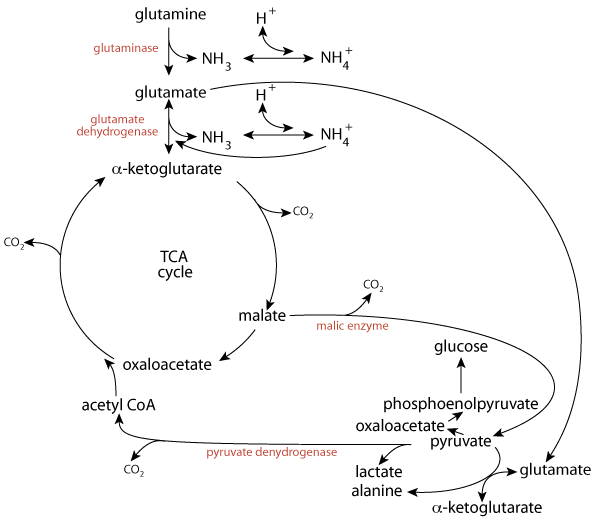
Excess nitrogen could be donated in a transamination reaction to pyruvate, converting it to alanine, which could carry the excess nitrogen to the liver for disposal in urea.
Fate of α-Ketoglutarate In The Kidney
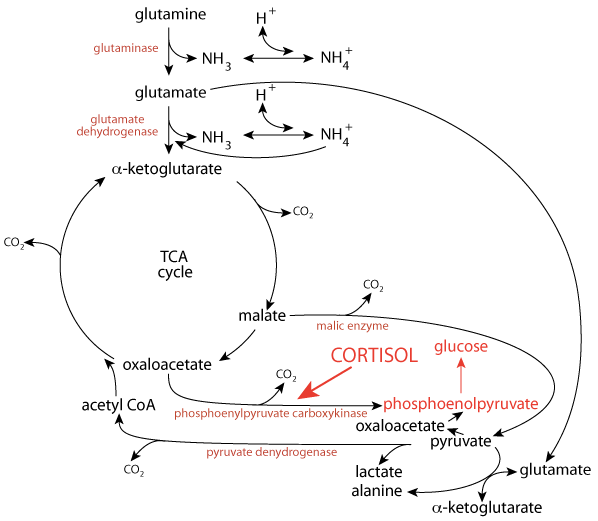
Cortisol, a glucocorticoid — it causes glucose levels to raise — secreted in response to chronic stress, induces the transcription of phosphoenolpyruvate carboxykinase (in both the kidney and the liver), which converts oxaloacetate to phosphoenolpyruvate that is used for the synthesis of glucose.
NOTE: cells of the renal medulla have a relatively high dependence on anaerobic glycolysis due to their lower oxygen supply and mitochondrial capacity; the lactate released from anaerobic glycolysis in these cells is taken up and oxidized in the renal cortical cells, which have a higher mitochondrial capacity and greater blood supply.
Click The Image
Cortisol Activation of PEPCK Gene Transcription
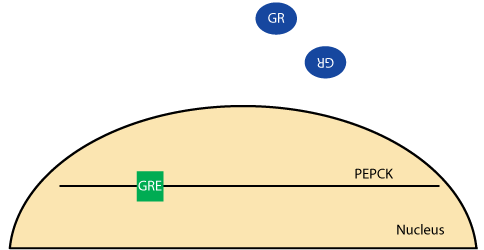
The unliganded glucocorticoid (GR) receptor is located in the cytosol and inactive.
Glucocorticoid Receptor: Cortisol Binds
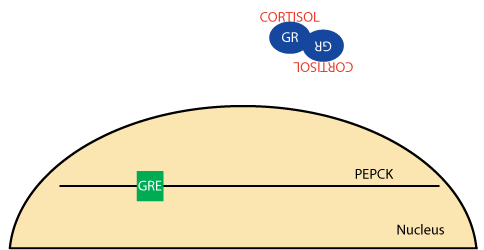
Cortisol, a glucocorticoid, binds the glucocorticoid receptor, causing it to dimerize and become active.
Glucocorticoid Receptor: Transfer to Nucleus
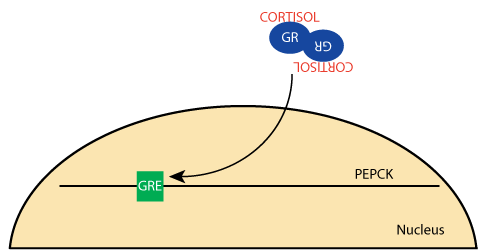
The active glucocorticoid receptor bound to cortisol enters the nucleus and binds to the Glucacorticoid Response Element [GRE] in glucocorticoid-regulated genes.
Glucocorticoid Receptor: PEPCK Gene Activation
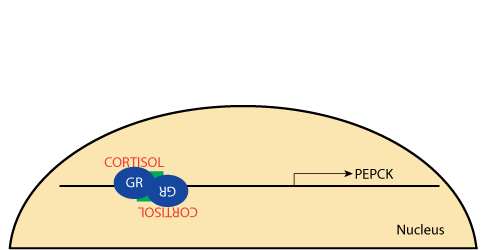
The gene for phosphophoenylpyruvate carboxykinase contains a glucacorticoid response element that binds the liganded glucicorticoid receptor, which activates its transcription in both the kidney and liver, causing glucose synthesis in both tissues (gluconeogenesis).
Liver
The liver is the major site of amino acid catabolism. It converts amino acid ammonia, ammonium ion, to urea for excretion. Normally it converts most of the amino acid carbon skeletons to intermediates of the TCA cycle or pyruvate, which are major source of its energy. During prolonged hypoglycemia, the liver derives a major source of its energy from the β-oxidation of fatty acids. The liver uses the acetyl CoA end product of β-oxidation to synthesize ketone bodies, which it releases into the blood as a source of energy for extra-hepatic tissues that contain mitochondria, particularly the brain. Amino acid carbon skeletons generated in the liver during hypoglycemia are not required for liver energy generation, but instead become available for the synthesis of glucose.
Glucagon signaling in the liver results in an increase in the number of liver amino acid transporters, particularly alanine transporters, allowing the liver to take an increased number of amino acids from the blood, which are supplied by the inreased protein degradation in extra-hepatic tissues in response to cortisol signaling. Glutamine is spared degradation in the liver because signaling by cortisol, which is secreted in response to several chronic stresses, including hypoglycemia and acidosis, downregulates glutaminase in the liver periportal cells. Thus, the glutamine, a significant nitrogen donor, becomes available to whichever cells respond to any particular chronic stress, with respect to acidosis, the kidney gets most of the glutamine, but, for examaple significant infections, cells of the immune system get most of the glutamine as the increase in number to eliminate infectious agents.